Smart Implantable Medical Systems (SIMS)
The SIMS (Smart Implantable Medical Systems) project is focused on the development of soft- and hardware for the next generation of neurostimulators and general implantable medical devices.
Neurostimulation is the electrical stimulation of the nervous system, which may be used for the treatment of many medical conditions. The advantage of electrical stimulation over pharmaceutical means is that the stimulation can be applied at specific locations, limiting the side-effects experienced by the patient. Examples of such treatments include chronic pain management through spinal-cord stimulation, inhibiting the tremor observed in Parkinson patients through deep-brain stimulation or preventing epileptic seizures through vagus-nerve stimulation. In addition to limiting the side-effects of the treatment, neurostimulation allows for treatments not previously possible, for example, allowing the deaf to hear using a cochlear implant.
Due to the pervasive nature of the aforementioned treatments, neurostimulators are implemented as wearable or even implantable devices. These are resource-constrained embedded devices, expected to perform a life-critical application on a small battery supply for several years. The treatment software running on these devices should be adjustable (e.g., to update a treatment plan) and monitored remotely, i.e., secured wireless communication is required.
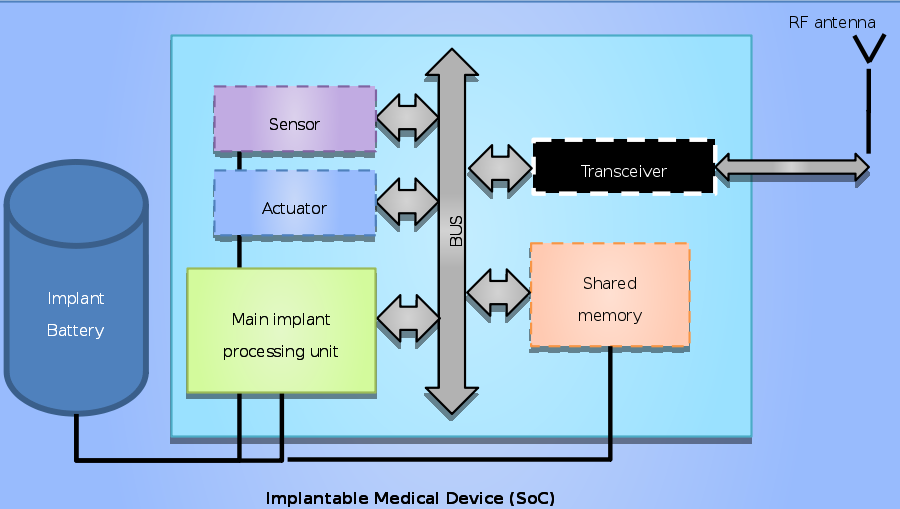
Given these concerns and constraints, we are currently working on the following topics within our department:
- Security of wireless communication to prevent malicious third parties from harming a patient using the wireless link, e.g., by stealing private data or altering device configurations;
- Rapid prototyping of new medical treatments to improve current health care. Examples include a minimal-delay seizure-prevention treatment and tinnitus treatment;
- Ultra-low-power and energy consumption to maximize the device lifetime; and
- Fault diagnosis and tolerance to guarantee correct functionality throughout a device’s lifetime, while minimizing the effect on the energy and power consumption.
Publications
For SiMS-related publications, please visit our Publications page.